Ductile fracture is a phenomenon that is of paramount importance in the field of materials science and engineering, especially within the realm of ocean engineering. This type of fracture is characterized by extensive plastic deformation before the actual separation of the material. Unlike brittle fracture, which occurs suddenly and without warning, ductile fracture provides ample indication through noticeable deformation, allowing for preventive measures to be taken before catastrophic failure. This blog delves into the mechanics of ductile fracture, its significance in ocean engineering, and how understanding this phenomenon can lead to the development of safer, more reliable ocean structures.
Mechanics of Ductile Fracture
Ductile fracture occurs in stages, beginning with the nucleation of voids within the material. These voids typically form around inclusions or second-phase particles that are inherently present within the metal or alloy. As the material undergoes stress, these voids grow and eventually coalesce, leading to the formation of a crack. The crack propagates through the material, causing eventual separation.
The process of ductile fracture can be broken down into three distinct stages:
- Nucleation: The initial formation of microscopic voids around particles or inclusions within the material.
- Growth: The voids increase in size as the material continues to deform under stress.
- Coalescence: The voids merge, forming a crack that leads to the final fracture of the material.
Ductile Fracture in Ocean Engineering
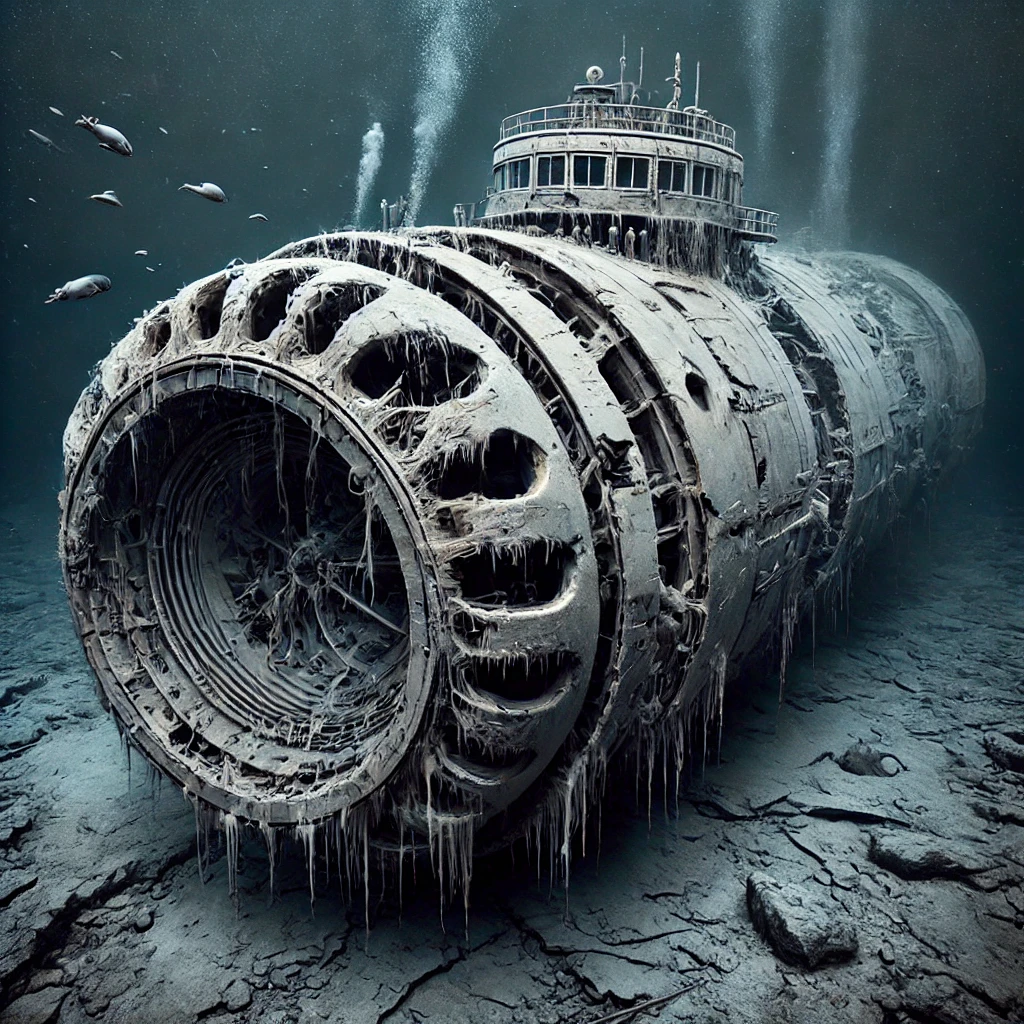
In the context of ocean engineering, ductile fracture is a critical consideration due to the harsh and often unpredictable conditions that ocean structures must withstand. Structures such as offshore platforms, subsea pipelines, and ships are regularly subjected to severe mechanical stresses, corrosive environments, and fluctuating temperatures. Understanding ductile fracture and its implications is essential for designing structures that can endure these challenges without catastrophic failure.
Offshore Platforms
Offshore platforms, whether fixed or floating, are massive structures designed to extract oil and gas from beneath the ocean floor. These platforms must endure the relentless forces of waves, currents, and winds. A failure in any component due to ductile fracture can have severe consequences, including environmental disasters and loss of human life. Engineers must ensure that the materials used in constructing these platforms exhibit high ductility, allowing for significant deformation before failure, thus providing a safety buffer against sudden collapse.
Subsea Pipelines
Subsea pipelines are the lifelines that transport oil and gas from offshore platforms to onshore facilities. These pipelines are laid on the ocean floor and are exposed to high pressures, corrosive seawater, and mechanical impacts from underwater debris. Ductile fracture is particularly relevant for these pipelines as it allows for some degree of deformation before rupture, which is crucial for maintaining the integrity of the pipeline. Engineers use materials and coatings that enhance the ductility and corrosion resistance of these pipelines to prevent failures that could lead to massive spills and environmental damage.
Ships and Submarines
Ships and submarines navigate through some of the most challenging environments on Earth. The hulls of these vessels are subjected to constant mechanical stresses from waves and impacts with floating debris. Ductile fracture plays a significant role in ensuring the structural integrity of these vessels. Materials with high ductility can absorb considerable energy before fracturing, which is vital for the safety of the crew and the vessel. Understanding ductile fracture mechanics allows engineers to design hulls that are not only strong but also resilient to the rigors of ocean travel.
Advances in Material Science
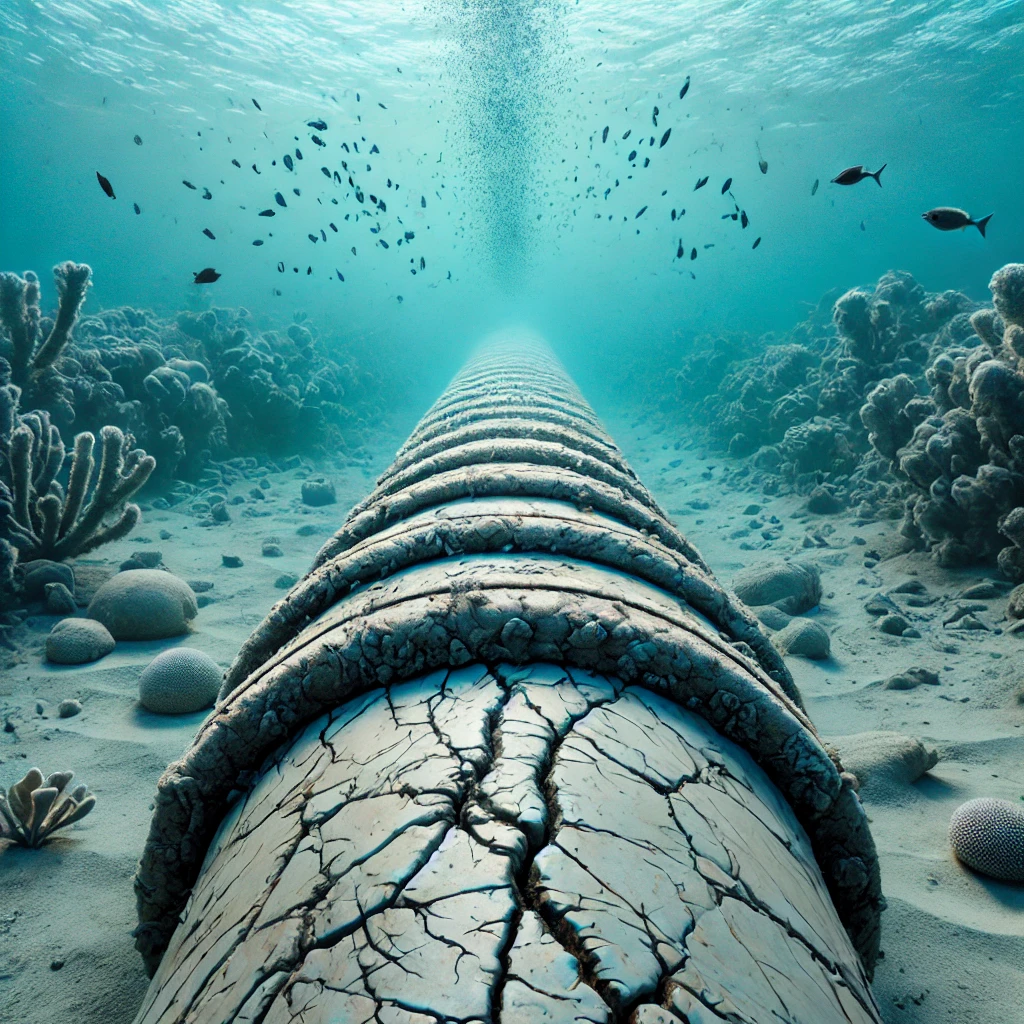
The study of ductile fracture has led to significant advances in material science, particularly in the development of new alloys and composites that exhibit superior ductility. For instance, high-strength low-alloy (HSLA) steels are widely used in ocean engineering applications due to their excellent combination of strength and ductility. These steels can undergo substantial plastic deformation before fracturing, making them ideal for use in structures that are subject to high stresses.
Additionally, the development of corrosion-resistant alloys has been a game-changer for ocean engineering. Materials such as duplex stainless steels and titanium alloys offer high ductility along with excellent resistance to seawater corrosion. These materials are commonly used in the construction of subsea pipelines and other underwater structures, where both mechanical strength and corrosion resistance are crucial.
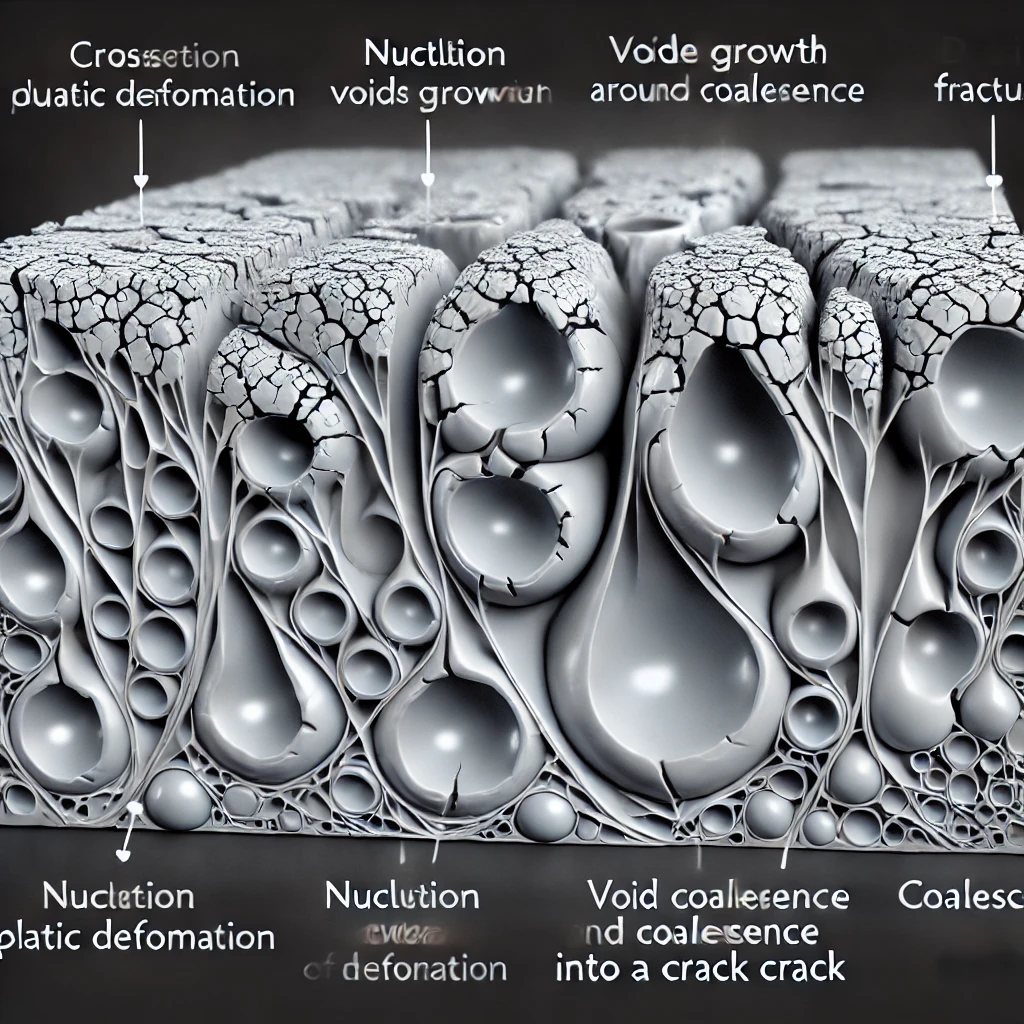
Simulation and Modeling
Advances in computational modeling and simulation have also enhanced our understanding of ductile fracture. Finite element analysis (FEA) allows engineers to simulate the behavior of materials under various stress conditions, providing insights into how ductile fracture might occur in real-world scenarios. These simulations can predict the initiation and propagation of cracks, helping engineers design structures that are more resistant to ductile fracture.
For example, in the design of offshore platforms, FEA can be used to model the stresses and strains that different components will experience. By understanding where and how ductile fracture is likely to occur, engineers can reinforce these areas with additional material or design modifications that mitigate the risk of failure.
Case Studies in Ocean Engineering
Several case studies highlight the importance of understanding ductile fracture in ocean engineering. One notable example is the failure analysis of the Alexander L. Kielland platform, a semi-submersible drilling rig that capsized in 1980, resulting in the loss of 123 lives. The investigation revealed that ductile fracture played a significant role in the collapse of the platform. The lessons learned from this disaster have led to stricter regulations and improved design practices to prevent similar failures in the future.
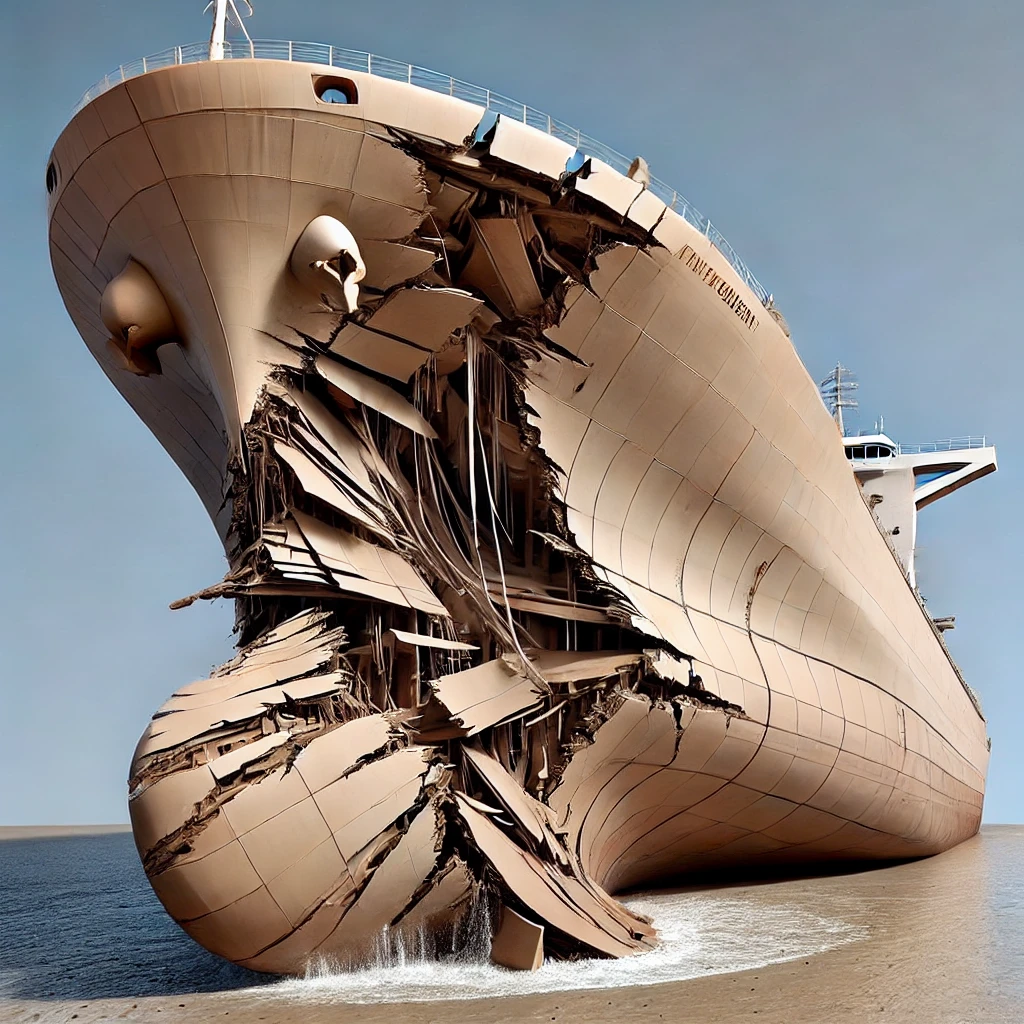
Another example is the development of deep-sea submersibles, which operate at extreme depths and pressures. The materials used in these submersibles must exhibit high ductility to withstand the immense forces encountered at such depths. Research into ductile fracture has been instrumental in selecting and developing materials that can ensure the safety and reliability of these advanced underwater vehicles.
Ductile fracture is a critical concept in materials science and ocean engineering, providing a safety net through plastic deformation before catastrophic failure. Understanding the mechanics of ductile fracture and its implications in ocean engineering can lead to the development of safer, more reliable structures capable of withstanding the harsh marine environment. From offshore platforms and subsea pipelines to ships and submarines, the principles of ductile fracture guide the design and material selection processes, ensuring the longevity and integrity of these essential structures. Advances in material science and computational modeling continue to enhance our ability to predict and prevent ductile fracture, paving the way for a safer and more resilient ocean engineering industry.