Autonomous Underwater Vehicles (AUVs) represent a convergence of advanced engineering and computational technologies. These vehicles, capable of performing tasks without direct human intervention, are indispensable in deep-sea exploration, environmental monitoring, and industrial inspections. AUV design demands an intricate balance between energy efficiency, maneuverability, structural integrity, and noise reduction.
Among the key tools shaping modern AUVs is Computational Fluid Dynamics (CFD). Through mathematical modeling and numerical simulation of fluid flow, CFD allows researchers and engineers to optimize AUV hull shapes, propulsion systems, and overall hydrodynamic performance. This blog explores in detail how CFD is transforming the design of AUVs, focusing on hull design, energy optimization, noise reduction, and adaptive navigation strategies.
1. Hull Design Optimization
Hydrodynamics and the Role of CFD
The hull’s shape directly impacts an AUV’s performance by determining drag, lift, and stability. CFD is indispensable in evaluating these factors, as it enables the simulation of fluid-structure interactions under various operational conditions.
Key CFD Techniques in Hull Design:
- Streamlined Hulls: Using CFD, engineers refine hull geometries to achieve laminar flow, reducing drag forces.
- Pressure Distribution Analysis: By simulating underwater pressure fields, CFD identifies zones of high drag or flow separation.
- Vortex Shedding Studies: CFD tools such as Large Eddy Simulation (LES) help mitigate vortex-induced vibrations, improving stability.
Example:
For a torpedo-shaped AUV, CFD simulations demonstrated a 25% drag reduction after iterative geometry adjustments, enabling extended mission durations.
Integration of Appendages
While appendages such as fins, rudders, and sensors are essential, they disrupt flow patterns, increasing drag. CFD allows precise analysis of their placement to minimize flow disturbances.
Design Strategies via CFD:
- Optimizing fin shapes and angles for better maneuverability.
- Reducing turbulence generated by sensor protrusions.
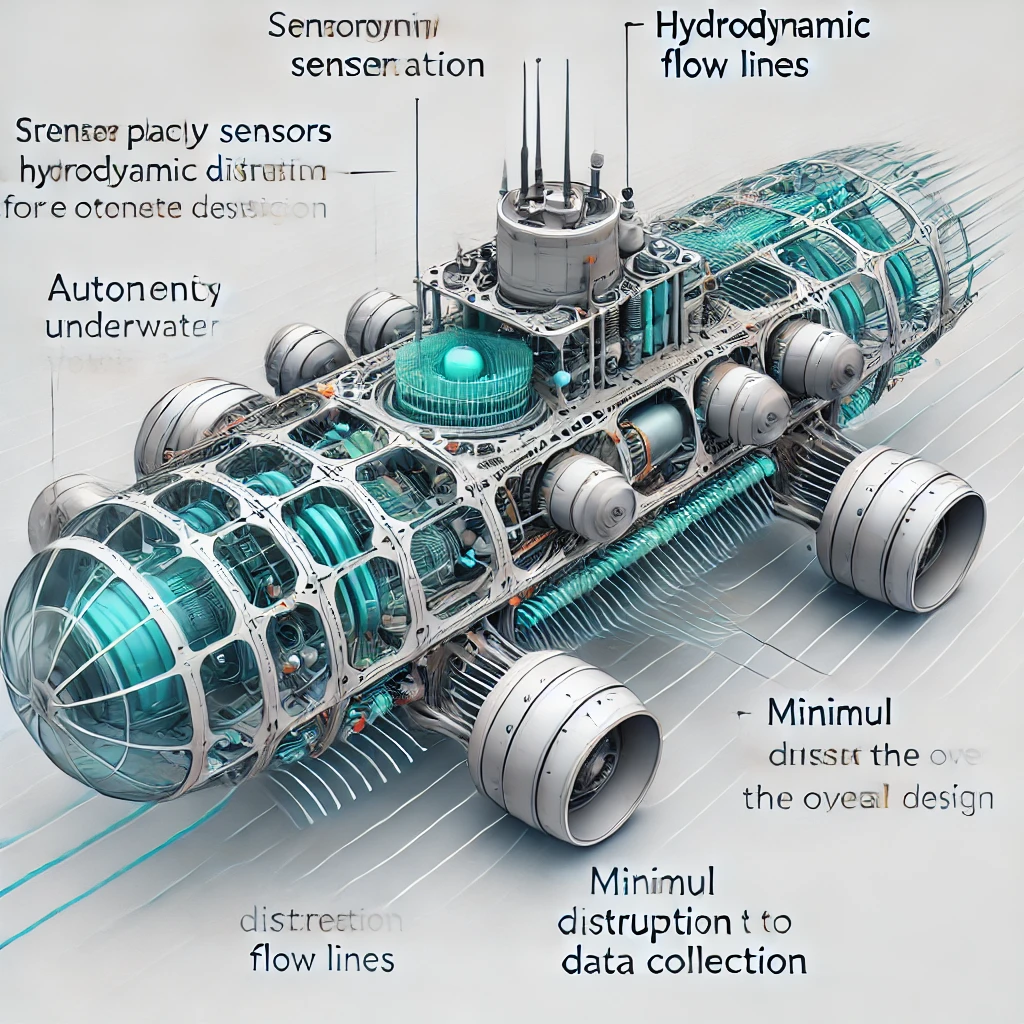
2. Power Systems Optimization
Energy Efficiency
Energy efficiency in AUVs is pivotal, as battery capacity often limits mission duration. Propulsion systems consume the most energy, making their optimization a critical area of research. CFD assists in:
- Analyzing propeller flow to minimize cavitation.
- Balancing thrust with drag forces for optimal power usage.
Case Study:
A CFD-driven design reduced propeller cavitation in a deep-sea AUV, extending battery life by 15% without compromising propulsion efficiency.
Propulsion and Flow Interactions
Advanced CFD techniques, such as Reynolds-Averaged Navier-Stokes (RANS) modeling, enable:
- Prediction of wake flow effects behind the hull.
- Optimization of thruster placement for even power distribution.
Renewable Energy Integration:
Some AUVs incorporate solar panels or wave-energy converters. CFD ensures that these systems are designed to interact harmoniously with water flow, maintaining the overall hydrodynamic profile.
3. Noise Reduction for Stealth and Environmental Safety
Sources of Noise
Noise reduction is crucial in both ecological and military contexts. Two primary noise sources in AUVs are:
- Hydrodynamic Noise: Caused by turbulent flow around the hull.
- Propeller Noise: Resulting from cavitation and blade-vortex interactions.
CFD-Driven Noise Mitigation
CFD simulations allow engineers to:
- Predict acoustic signatures through Pressure-Velocity Coupling (P-V coupling).
- Design propeller blades with minimal cavitation zones.
- Optimize hull coatings to reduce frictional drag, indirectly decreasing noise.
Example:
Using LES, a team redesigned an AUV propeller to achieve a 40% reduction in cavitation-induced noise, improving stealth capabilities for surveillance missions.
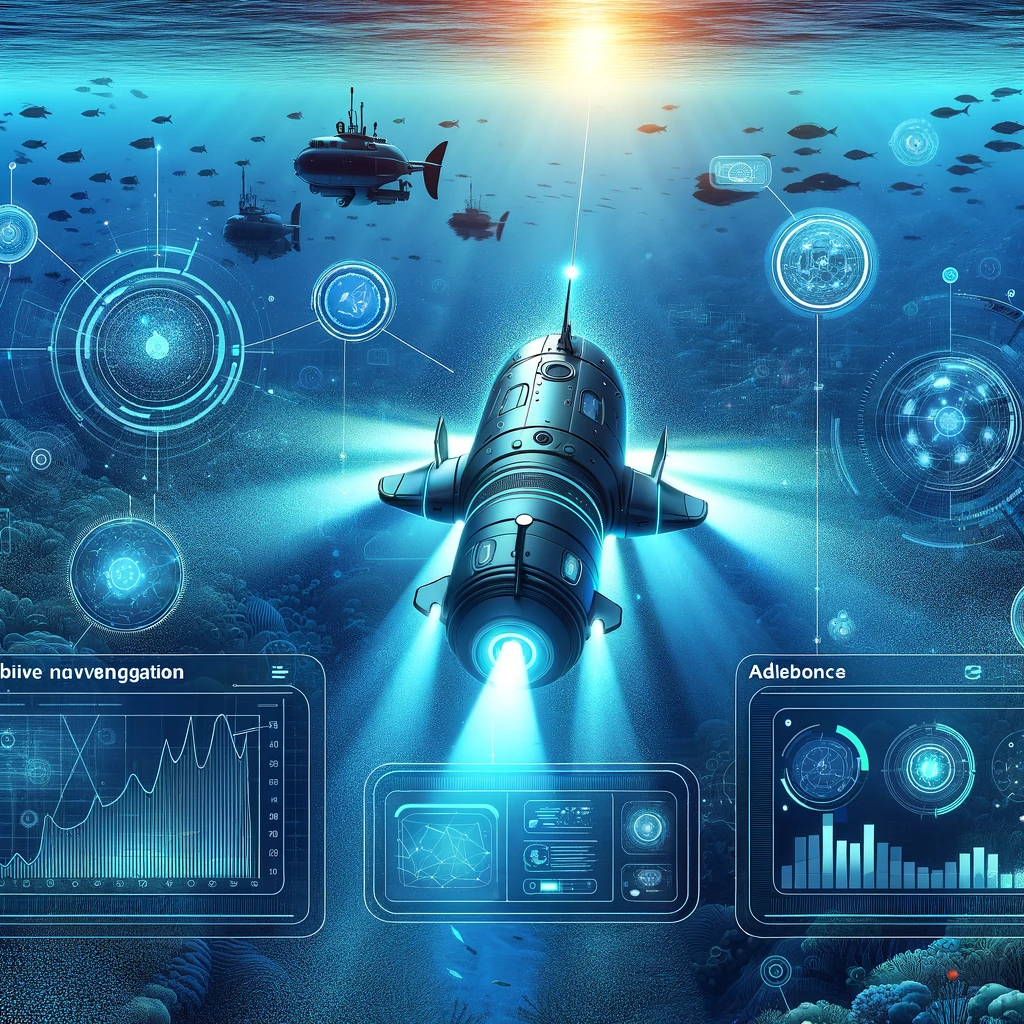
4. Adaptive Control and AI-Based Navigation
Role of AI in Navigation
Artificial Intelligence (AI) enables AUVs to adapt dynamically to changing underwater environments. However, successful navigation depends on accurate hydrodynamic models, which CFD provides.
Applications of CFD in AI-Based Navigation:
- Modeling current patterns for real-time path planning.
- Integrating flow field data with reinforcement learning algorithms for obstacle avoidance.
Example:
CFD-informed AI navigation helped an AUV adjust its trajectory in turbulent currents, reducing energy consumption by 18% during a 12-hour mission.
5. Sensor Integration and Placement
Challenges in Sensor Placement
Sensors such as sonar, cameras, and environmental monitors are integral to AUV functionality. However, their integration can increase drag and alter flow patterns. CFD addresses these challenges by:
- Identifying low-drag zones for sensor placement.
- Simulating the effect of sensor housing shapes on overall hydrodynamics.
Dynamic Sensor Pods
Some AUVs use retractable sensor pods. CFD ensures these pods operate smoothly, minimizing flow disruptions when deployed or retracted.
6. Advances in Computational Fluid Dynamics
High-Fidelity Simulations
The increasing power of high-performance computing has elevated CFD simulations, enabling:
- Realistic modeling of turbulence using DNS and LES techniques.
- Multi-phase flow simulations for environments with varying salinity and temperature.
CFD in Multi-Objective Optimization
Combining CFD with optimization algorithms enables engineers to achieve conflicting goals, such as drag reduction and noise minimization. Genetic algorithms and machine learning models are now integrated with CFD simulations for this purpose.
Example:
A multi-objective optimization study improved both energy efficiency (by 10%) and stealth (by 15%) in a next-generation AUV.
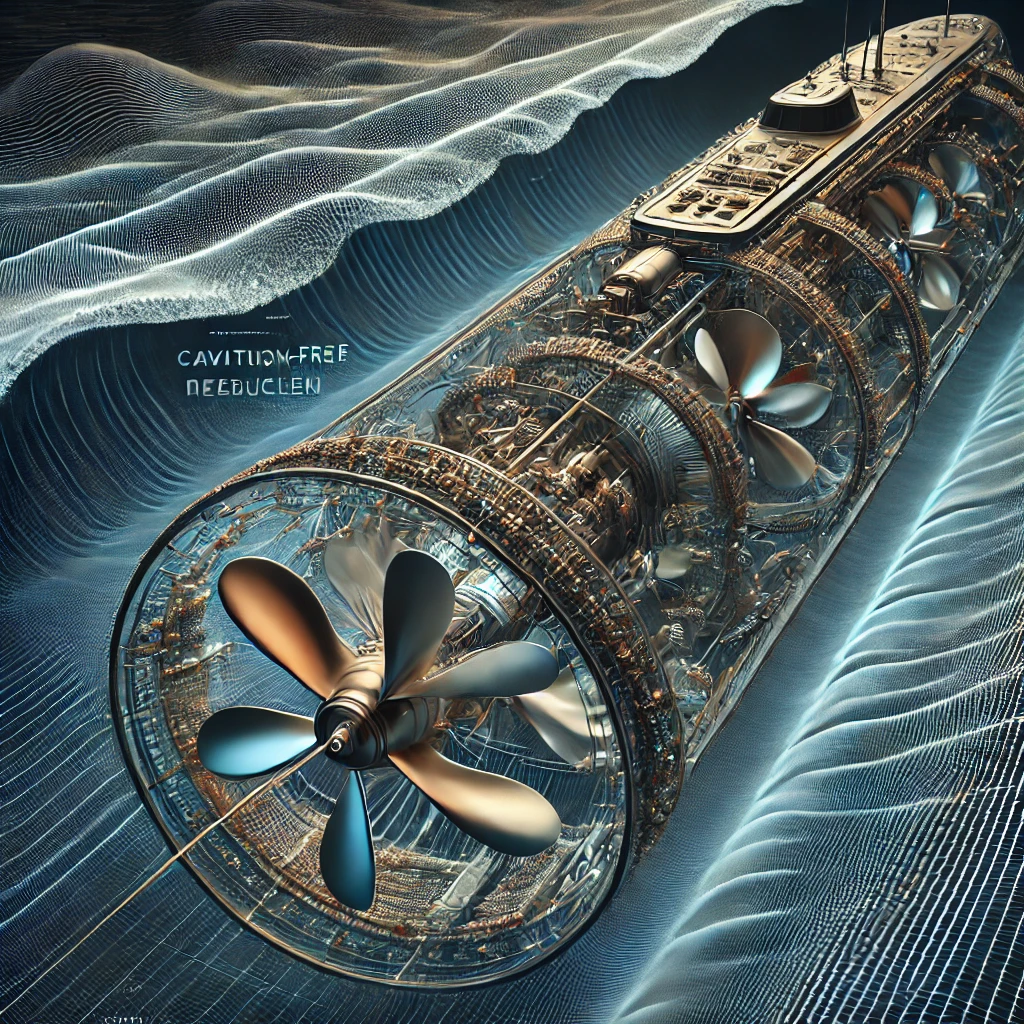
7. Enhancing Mission Capabilities Through CFD-Informed Design
AUVs are not just technical marvels but also mission-specific tools that must meet the unique demands of diverse underwater tasks. Computational Fluid Dynamics (CFD) enhances these mission capabilities by tailoring AUV designs to specific operational environments and objectives.
Customizing Hull Design for Diverse Missions
CFD empowers engineers to optimize AUVs for various underwater applications, including exploration, scientific research, resource extraction, and surveillance. Each mission imposes unique constraints on the vehicle’s design and functionality.
- Deep-Sea Exploration
- Challenge: Operating at extreme depths with high pressure and low temperatures requires robust hulls that minimize drag and resist structural deformation.
- CFD Contribution: High-fidelity simulations predict the performance of hull materials under these harsh conditions, ensuring structural integrity while maintaining hydrodynamic efficiency.
- Example: Using CFD, researchers developed titanium-based hull designs for an AUV tasked with exploring hydrothermal vents, achieving a 30% improvement in drag resistance under high-pressure conditions.
- Surveillance Missions
- Challenge: Stealth and long-duration performance are critical for military and monitoring tasks.
- CFD Contribution: CFD simulations refine hull shapes and propeller designs to reduce acoustic signatures, ensuring low detectability. Flow analysis is also used to optimize battery usage, extending mission duration.
- Example: A surveillance AUV designed with CFD achieved an operational duration increase of 15% by reducing flow noise and improving energy efficiency.
- Scientific Sampling and Data Collection
- Challenge: Deploying and recovering sensor arrays, sampling arms, and other equipment adds drag and turbulence.
- CFD Contribution: Simulations model the impact of deployed equipment on flow dynamics, ensuring the vehicle remains stable during data collection operations.
- Example: A multi-sensor AUV used in coral reef studies integrated CFD-optimized appendages, reducing flow disturbances and enabling higher-resolution imaging.
Optimizing for Currents and Turbulence
Underwater currents and turbulence are major challenges for AUVs, particularly in dynamic environments like coastal waters or tidal zones. CFD enables the design of adaptive AUV systems capable of maintaining performance in such conditions.
- Real-Time Flow Adaptation
- CFD-based flow models inform AI algorithms, enabling real-time adjustments to propulsion and control surfaces.
- Example: A tidal-monitoring AUV utilized CFD data to predict and counteract current-induced drifts, maintaining a stable position during long-term monitoring.
- Turbulence Mitigation
- LES and DES simulations help predict turbulent flow regions, allowing for better control surface designs that stabilize the vehicle.
- Example: By using CFD-informed turbulence predictions, an offshore pipeline inspection AUV maintained stability even in vortex-prone environments, ensuring accurate data collection.
Payload Integration and Modular Designs
AUVs often carry specialized payloads, including sonar systems, cameras, and robotic arms. Payload integration presents a significant design challenge, as it impacts the vehicle’s hydrodynamic and energy performance.
- Dynamic Payload Configurations
- Modular payloads allow AUVs to switch between different configurations. CFD simulations are used to model the hydrodynamic effects of each configuration, ensuring performance optimization regardless of the payload.
- Example: A research AUV deployed in Arctic waters optimized its hydrodynamic profile using CFD to accommodate both imaging sensors and sampling arms.
- Improving Sensor Functionality
- Sensors mounted on the hull can experience flow-induced vibrations that degrade data quality. CFD simulations help identify low-disturbance zones for sensor placement.
- Example: A CFD-optimized AUV achieved 20% higher sonar resolution by minimizing sensor vibration.
Environmental Sustainability
AUV design is increasingly being shaped by the need to reduce environmental impacts, particularly in fragile ecosystems. CFD aids in achieving sustainability by:
- Designing quieter AUVs to minimize disturbance to marine life.
- Improving energy efficiency to reduce the frequency of battery replacements and vehicle retrieval.
Example:
An AUV designed for marine conservation used CFD to reduce noise levels by 50%, allowing it to operate in ecologically sensitive areas without disrupting local fauna.
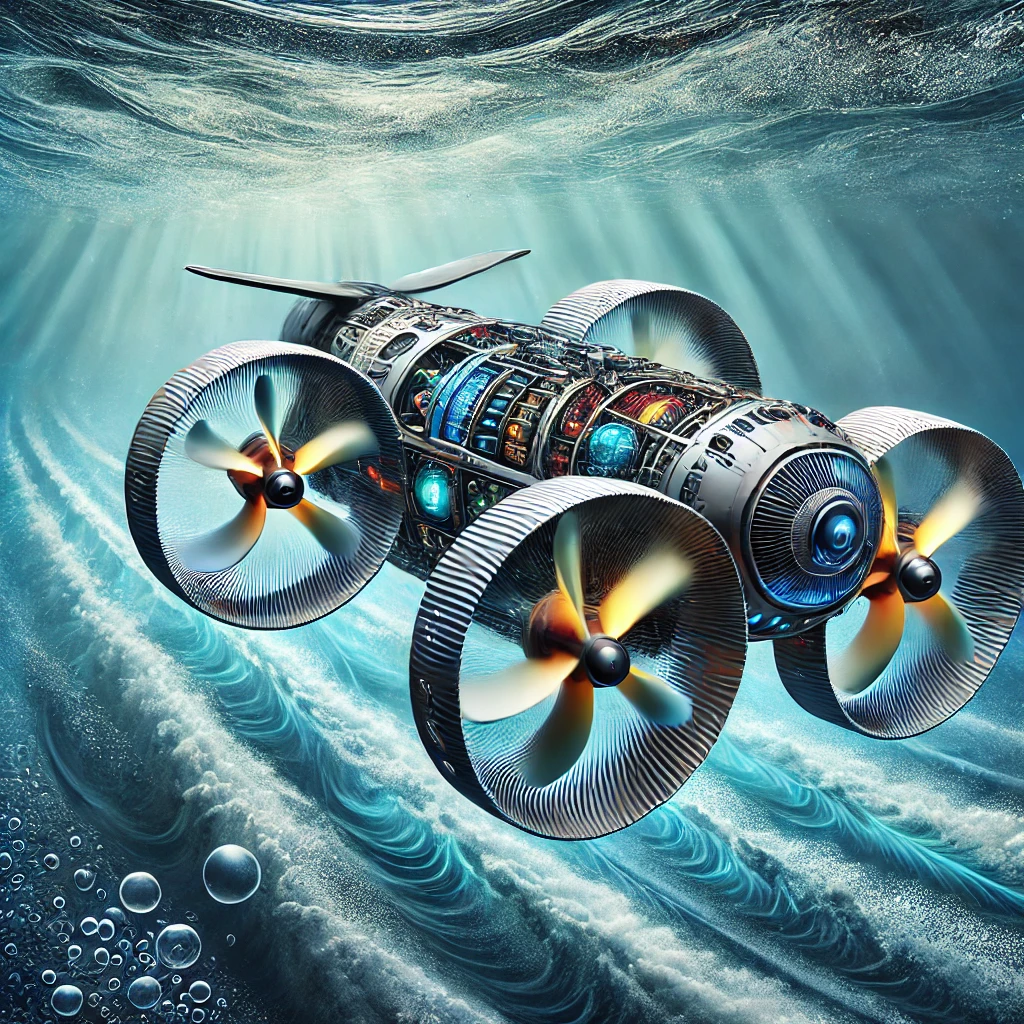
8. Future Directions in AUV Design
Digital Twins
Digital twins integrate real-time data with CFD simulations to provide dynamic feedback during AUV operations. This technology allows adaptive designs that can respond to environmental changes mid-mission.
Additive Manufacturing
CFD-guided designs can be directly implemented through 3D printing, enabling rapid prototyping of hydrodynamic hulls with complex geometries.
Quantum Computing for CFD
Quantum algorithms hold the promise of accelerating CFD calculations, allowing near-instantaneous modeling of complex underwater flows.
Conclusion
The synergy of Computational Fluid Dynamics, advanced power systems, noise reduction strategies, and AI-based navigation is driving the evolution of AUVs. As CFD tools continue to advance, they are unlocking unprecedented opportunities to design AUVs that are efficient, robust, and capable of navigating the harshest underwater environments.
From enhancing hydrodynamic efficiency to enabling renewable energy integration, CFD remains at the heart of AUV innovation. Its applications span every aspect of design and operation, making it indispensable for the next generation of autonomous underwater exploration.